Being a heterogeneous disease, acute myeloid leukemia (AML) is highly variable in cytogenetic and molecular characteristics, which are considered important prognostic factors during diagnosis, and play an essential role in risk stratification and clinical decision making.1,2 Adult AML patients can achieve hematological complete remission (CR) with bone marrow blasts < 5%,3 through intensive chemotherapy regimens. Nevertheless, most of these patients, about 60% to 70%,4 will eventually relapse.5 The persistence of leukemic cells after chemotherapy unidentified by routine morphologic evaluation is known as minimal residual disease (MRD),6 and is the main cause of relapse.4
The abnormal expression of immunophenotypic markers that distinguishes the leukemic cells is known as leukemia-associated immunophenotypes (LAIPs),7 which are not present or very infrequently present on normal bone marrow cells.6,8,9 LAIPs in AML include asynchronous antigen expression, cross-lineage antigen expression, antigen under/overexpression, and absence of lineage-specific antigens.6,7 LAIPs can be used to determine MRD by multiparameter flow cytometry (MFC).5,6 Detection of LAIPs varied from 50% to almost 100%, depending on different laboratories and protocols.5,6 No standard method has been established using MFC. Recently some recommendations were published in a consensus document from the European LeukemiaNet (ELN) MRD Working Party.10
The change in aberrant immunophenotype during disease progression imposes a technical difficulty and limitation for MRD detection using LAIPs by MFC, which limits its diagnostic performance and explains the high rate of relapse associated with MRD negative patients.11 Increasing the number of fluorochromes (at least eight) in one tube allows identification of a higher number of LAIPs thus improving the performance of MRD analysis using this strategy.12,13
Alteration of LAIPs can occur during disease relapse.5,14–16 This suggests that relapse might be due to either clonal evolution or the occurrence of secondary AML due to chemotherapy treatment effect.5 However, in most of these studies, the detection of immunophenotype changes was focused on the subgroup of patients who displayed highly aberrant phenotypes, which comprise 60% to 80% of all AML cases.15 In light of this data, we used an eight-color MFC panel to identify LAIPs in 50 AML patients at diagnosis and to detect if they showed any alteration in relapsed/refractory cases.
.png)
Figure 1: The gating strategy used to identify leukemia-associated immunophenotypes. (a) Identification of blasts with the help of CD45/ side scatter (SSC) gating strategy depending on dim expression of the CD45 antigen and being low SSC (P1). Gating on lymphocytes was done as an internal control (P6/green in color). (b) Low forward scatter (FSC) (non-viable cells and erythroid cells) within the FSC/SSC plot were then excluded (P2). (c) Sequential-gating strategy using immature/primitive markers CD34 and CD117 (P3). (d) Plotting of CD117 against myeloid marker CD33 (P4) showing that our (e) target population is CD13/CD7 double-positive (orange), while lymphocytes are CD7 positive only (green).
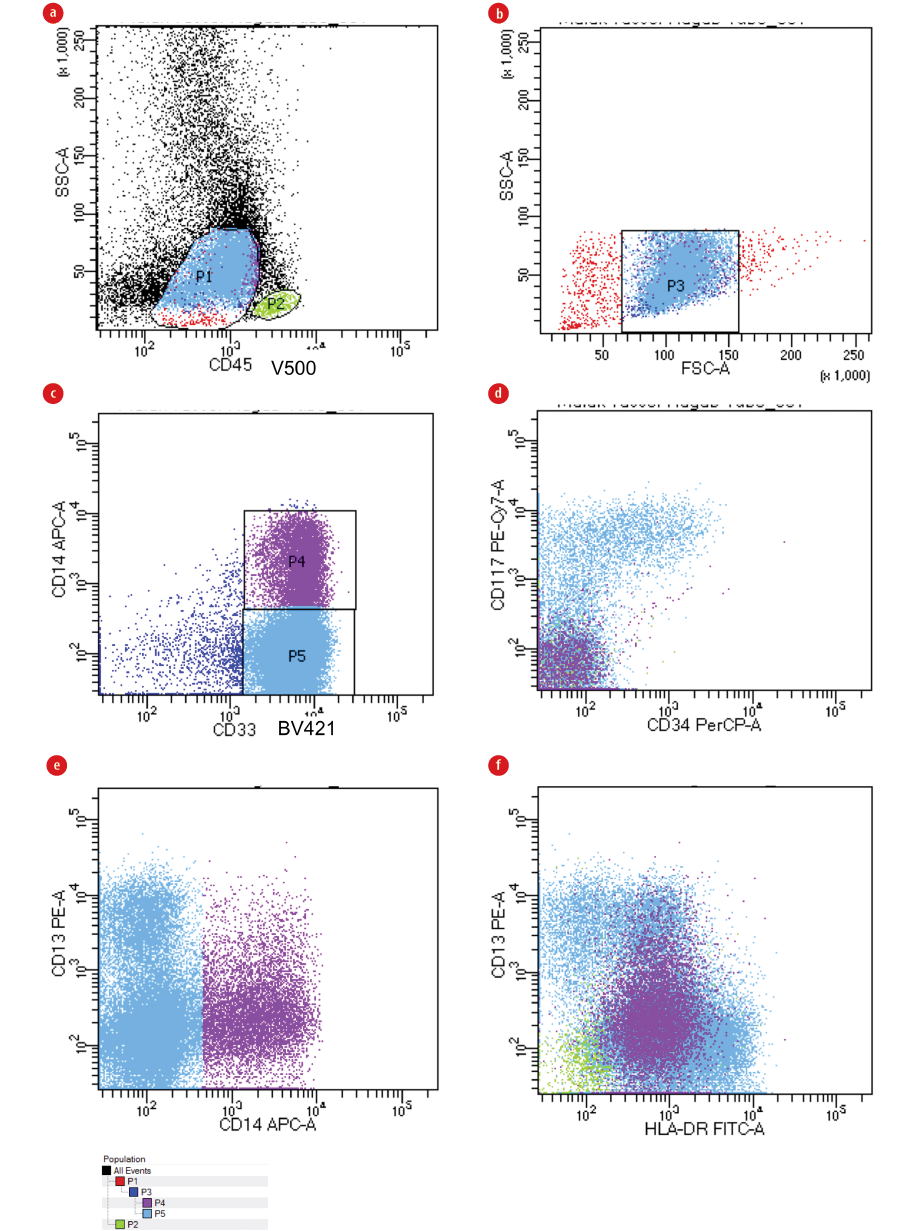
Figure 2: Gating strategy in CD34negative/CD117negative AML. (a) Identification of blasts with the help of CD45/ side scatter (SSC) gating strategy depending on dim expression of the CD45 antigen and being low SSC (P1). Gating on lymphocytes was done as an internal control (P2/green color). (b) Low forward scatter (FSC) (non-viable cells and erythroid cells) within the FSC/SSC plot were then excluded (P3). (c) An exclusion marker that has to be absent on the leukemic cells is used to exclude monocytes and/or granulocytes,20 in this case CD14 is used to exclude monocytes (P4) from blasts (P5). (d) Blasts are both CD34 and CD117 negative. (e and f) Show how CD14 differentiates monocytes (purple color) from blasts (blue color).
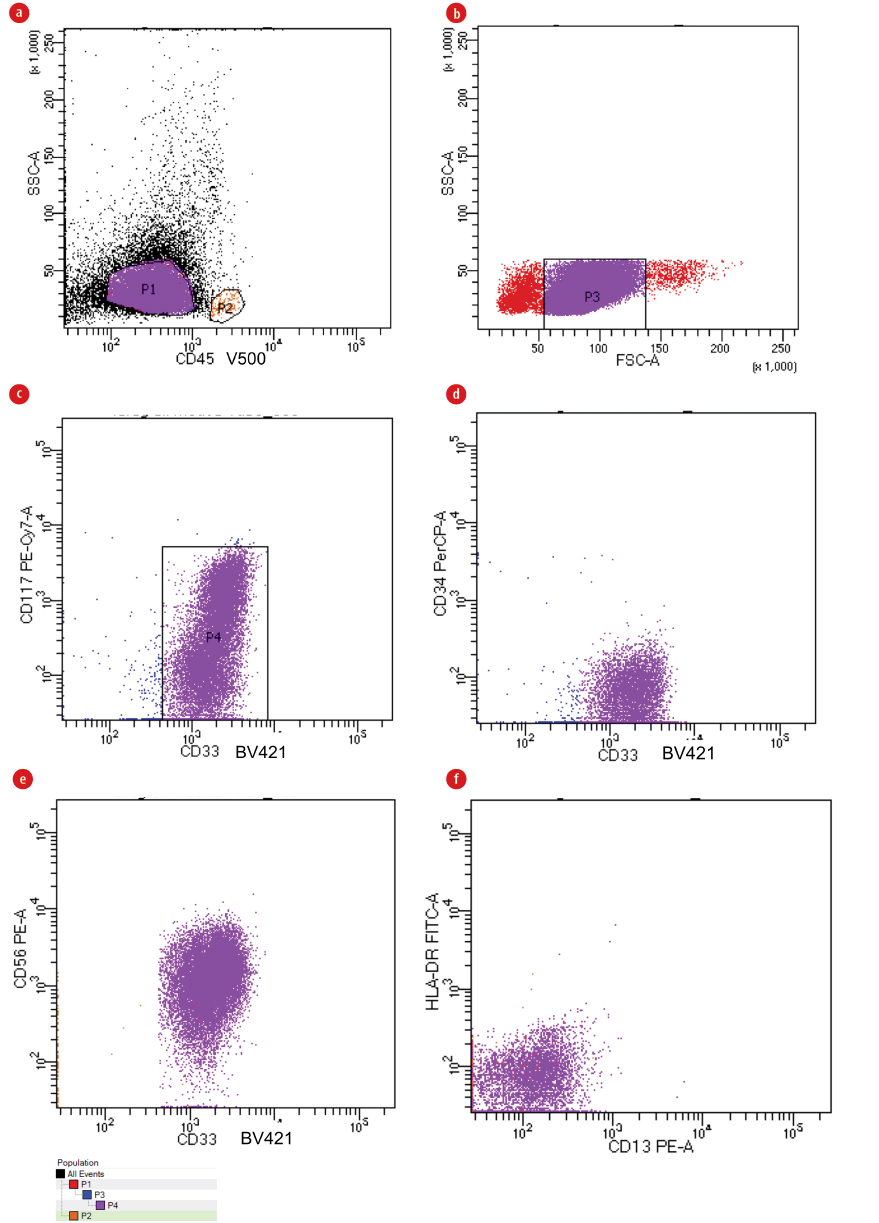
Figure 3 : (a) Identification of blasts with the help of CD45/ side scatter (SSC) gating strategy depending on the dim expression of the CD45 antigen and being low SSC (P1). Gating on lymphocytes was done as an internal control (P2/orange color/ very minimal in amount). (b) Low forward scatter (FSC) (non-viable cells and erythroid cells) within the FSC/SSC plot were then excluded (P3). (c) Sequential-gating strategy using immature/primitive marker CD117 against CD33 as the case is CD34 negative (P4). (d) Plotting of CD33 against CD34 to demonstrate that blasts are CD34 negative. (e) Our target population is CD33/CD56 double-positive (purple color), and is also (f) both CD13 and HLA-DR negative (purple color), while lymphocytes are very minimal (orange color in both e and f).
Methods
The present study was conducted on fresh bone marrow samples obtained from 50 consecutive, unselected AML patients (de novo and secondary AML) at diagnosis. Twenty bone marrow samples from age and sex matched patients performing bone marrow aspirate for non-malignant hematological indications were included as controls to determine the specificity of LAIPs. The study was performed in Alexandria University Hospitals (AUHs), Egypt, from September 2019 to December 2020. Patients and controls gave written informed consent after explaining the purpose and investigational nature of the study and its potential risks. The study was carried out according to AUHs protocols and was approved by the Medical Ethics Committee of Alexandria Faculty of Medicine before it started. AML cases were diagnosed based on morphologic findings, immunophenotyping, and cytogenetics.1,17 Patients characteristics are shown in Table 1. The cytogenetic/molecular risk categories were defined according to ELN risk stratification by genetics.3 Refractory AML was defined as the inability to achieve CR following one or two cycles of standard combination chemotherapy. While relapsed AML was defined as any proof of disease recurrence after CR. CR was defined as < 5% blasts present in the bone marrow, and the absence of circulating blasts and blasts with Auer rods in addition to > 1 × 109/L neutrophils and > 100 ×109/L platelets in the peripheral blood.3
Fresh bone marrow samples were lysed according to the EuroFlow standard operating protocol for bulk lysis for MRD panels (see www.EuroFlow.org for Version 1.3, updated on 25 June 2018).18
The final volume of cells was counted using cell counter ADVIA 2021i (Siemens, Germany), and the concentration was adjusted to 20 000 cells/μL in 1 mL phosphate-buffered saline and well mixed by vortex. A 100 μL of the prepared sample solution was pipetted in each tube to be stained with all the fluorochrome-labeled monoclonal antibodies (MoAb)19 shown in Table 2 in the order of designed/screening panel shown in Table 3.
A minimum of 1 × 105 cells was acquired using BD FACSCanto™ II (BD biosciences, California, USA). Samples were analyzed using FACSDiva™ software v 8.0.2 (BD biosciences, California, USA).
Median percentages of LAIPs positive cells in AML and normal bone marrow were calculated and compared.
Results
Identification of blasts was done using CD45/side scatter (SSC) log gating strategy excluding low forward scatter (FSC) (non-viable cells and erythroid cells) within the FSC/SSC plot. Sequential-gating strategies using immature/primitive markers CD34 and CD117 were used to better define the blast population especially in monocytic leukemia cases where there is no clear-cut between blasts and monocytic population. CD14 back-gating was performed to exclude any monocytes, and consequently, the LAIPs were defined on blast populations. CD45/CD34/CD117 were used in addition to different myeloid and lymphoid markers in the eight-color combination for LAIPs detection. The gating strategy used to identify LAIPs is shown in Figure 1, also see Figure 2 for gating strategy in CD34negative/CD117negativeAML, and Figure 3 for more examples of LAIPs.
Table 1: Patient characteristics.
Age, median (range), years |
28.5 (3.0–80.0) |
Gender, n (%) |
|
Male |
21 (42.0) |
Female |
29 (58.0) |
WBC, median (range), × 103/μL |
49.4 (0.7–362.3) |
Hemoglobin, median (range), g/dl |
8.0 (4.4–12.5) |
Platelets, median (range), × 103/μL |
31.5 (10.0–418.0) |
BM blasts, median (range), % |
79.5 (24.0–97.0) |
According to WHO classification,17 n (%) |
|
AML with recurrent genetic abnormalities |
|
AML with t(8;21)(q22;q22) |
2 (4.0) |
APL with PML-RARA |
2 (4.0) |
AML-MRC |
1 (2.0) |
AML, NOS |
|
AML with minimal differentiation |
1 (2.0) |
AML without maturation |
21 (42.0) |
AML with maturation |
2 (4.0) |
Acute myelomonocytic leukemia |
7 (14.0) |
Acute monoblastic and monocytic leukemia |
14 (28.0) |
FAB subtype, n (%) |
|
M0 |
1 (2.0) |
M1 |
21 (42.0) |
M2 |
4 (8.0) |
M3 |
2 (4.0) |
M4 |
8 (16.0) |
M5 |
14 (28.0) |
Cytogenetics/molecular risk stratification, n (%) |
|
Low risk |
5 (10.0) |
Intermediate risk |
36 (72.0) |
WBC: white blood cell; BM: bone marrow; WHO: World Health Organization; AML: acute myeloid leukemia; APL: acute promyelocytic leukemia; AML-MRC: AML with myelodysplasia-related changes; NOS: not otherwise specified; FAB: French-American-British classification.
Table 2: Monoclonal antibodies used in immunophenotyping.
HLA-DR |
TU36 |
FITC |
BD biosciences, California, USA |
CD15 |
HI98 |
FITC |
BD biosciences, California, USA |
CD22 |
HIB22 |
FITC |
BD biosciences, California, USA |
CD11c |
B-ly6 |
FITC |
BD biosciences, California, USA |
CD13 |
WM15 |
PE |
BD biosciences, California, USA |
CD133 |
W6B3C1 |
PE |
BD biosciences, California, USA |
CD56 |
B159 |
PE |
BD biosciences, California, USA |
CD11b |
ICRF44 |
PE |
BD biosciences, California, USA |
CD34 |
8G12 |
PerCP |
BD biosciences, California, USA |
CD117 |
104D2 |
PE-Cy7 |
BD biosciences, California, USA |
CD14 |
M5E2 |
APC |
BD biosciences, California, USA |
CD19 |
HIB19 |
APC |
BD biosciences, California, USA |
CD4 |
SK3 |
APC |
BD biosciences, California, USA |
CD7 |
M-T701 |
APC-H7 |
BD biosciences, California, USA |
CD2 |
RPA-2.10 |
APC-H7 |
BD biosciences, California, USA |
CD33 |
WM53 |
BV421 |
BD biosciences, California, USA |
FITC: fluorescein isothiocyanate; PE: phycoerythrin; PerCP: Peridinin-Chlorophyll-Protein; PE-Cy7: phycoerythrin-Cy7 tandem conjugate; APC: allophycocyanin; APC-H7: allophycocyanin-H7 tandem conjugate; BV421: brilliant violet 421; V500: violet 500.
Table 3: Designed/screening panel used for identifying leukemia-associated immunophenotypes at diagnosis.
1 |
HLA-DR |
CD13 |
CD34 |
CD117 |
CD14 |
CD7 |
CD33 |
CD45 |
2 |
CD15 |
CD133 |
CD34 |
CD117 |
|
CD2 |
CD33 |
CD45 |
3 |
CD22 |
CD56 |
CD34 |
CD117 |
CD19 |
|
CD33 |
CD45 |
FITC: fluorescein isothiocyanate; PE: phycoerythrin; PerCP: Peridinin-Chlorophyll-Protein; PE-Cy7: phycoerythrin-Cy7 tandem conjugate; APC: allophycocyanin; APC-H7: allophycocyanin-H7 tandem conjugate; BV421: brilliant violet 421; V500: violet 500.
Table 4: Frequency of LAIPs in 50 AML patients and healthy bone marrow.
Cross-lineage antigen expression (n = 41) |
CD34+/CD7+ |
12 |
7.0–96.6 |
0.01 (0.00–0.03) |
Good |
CD117+/CD7+ |
9 |
7.0–96.6 |
0.01 (0.00–0.03) |
Good |
CD117+/CD19+ |
8 |
8.0–82.0 |
< 0.01 (0.00–0.01) |
Strong |
CD34+/CD2+ |
5 |
8.0–92.0 |
< 0.01 (0.00–< 0.01) |
Strong |
CD117+/CD2+ |
4 |
8.0–92.0 |
< 0.01 (0.00–< 0.01) |
Strong |
CD34+/CD4+ |
1 |
89.3 |
< 0.01 (0.00–0.01) |
Strong |
CD117+/CD4+ |
1 |
82.8 |
< 0.01 (0.00–0.01) |
Strong |
CD33+/CD4+ |
1 |
94.8 |
< 0.01 (0.00–0.01) |
Strong |
Asynchronous expression (n = 58) |
CD34+/CD56+ |
8 |
20.0–79.0 |
< 0.01 (0.00–0.01) |
Strong |
CD117+/CD56+ |
8 |
28.0–87.1 |
< 0.01 (0.00–0.01) |
Strong |
CD33+/CD56+ |
9 |
17.0–97.0 |
< 0.01 (0.00–0.01) |
Strong |
CD34+/CD11b+ |
5 |
23.0–86.7 |
< 0.01 (0.00–0.09) |
Good |
CD117+/CD11b+ |
8 |
17.2–84.9 |
< 0.01 (0.00–0.09) |
Good |
CD34+/CD64+ |
6 |
13.0–91.0 |
< 0.01 (0.00–0.01) |
Strong |
CD117+/CD64+ |
11 |
14.0–95.0 |
< 0.01 (0.00–0.01) |
Strong |
CD34+/CD15+ |
2 |
30.1–52.0 |
< 0.01 (0.00–0.02) |
Good |
CD117+/CD15+ |
1 |
57.2 |
< 0.01 (0.00–0.02) |
Good |
Lack of antigens (n = 73) |
CD34+/CD13- |
2 |
14.6–98.2 |
0.04 (0.02–0.16) |
Weak |
CD34+/CD33- |
5 |
46.0–93.0 |
0.04 (0.01–0.16) |
Weak |
CD34+/HLA-DR- |
4 |
14.0–95.0 |
0.04 (0.01–0.28) |
Weak |
CD34+/CD117- |
4 |
9.0–96.6 |
0.34 (0.02–1.00) |
Weak |
CD117+/CD13- |
3 |
28.0–92.0 |
0.06 (0.02–0.16) |
Weak |
CD117+/CD33- |
5 |
41.0–91.0 |
0.05 (0.01–0.12) |
Weak |
CD117+/HLA-DR- |
11 |
37.0–99.0 |
0.04 (0.01–0.28) |
Weak |
CD117+/CD34- |
21 |
13.3–99.0 |
0.31 (0.08–1.48) |
Weak |
CD33+/CD13- |
6 |
39.8–98.0 |
0.03 (0.01–0.08) |
Good |
LAIPs: leukemia-associated immunophenotypes; AML: acute myeloid leukemia; BM: bone marrow.
*Some cases have more than one LAIP.
a% of positive cells in AML BM is given as a range when more than one case was identified.
bMedian percentage of LAIPs (minimum-maximum) in control BM.
Table 5: Leukemia-associated immunophenotypes (LAIPs) at initial diagnosis and persistent/relapsed disease.
11 (Refractory) |
CD45(dim)/CD34+/CD117+/ CD13+/CD33- |
CD45(dim)/CD34+/CD117+/CD13+/CD33-
(No change) |
17 (Refractory) |
CD45(dim)/CD34+/CD117+/ CD13+/CD33-/CD19+ |
CD45(dim)/CD34+/CD117+/CD13+/CD33-/CD19+
(No change) |
22 (Refractory) |
CD45(dim)/CD34+/CD117+/ CD13+/CD33+/CD19+ |
CD45(dim)/CD34+/CD117+/CD13+/CD33+/CD19+
(No change) |
33 (Refractory) |
CD45(dim)/CD34-/CD117+/ CD13+/CD33+/HLA-DR- |
CD45(dim)/CD34-/CD117+/CD13+/CD33+/HLA-DR-
(No change) |
34 (Refractory) |
CD45(dim)/CD34+/CD117+/ CD13+/CD33+/CD56+ |
CD45(dim)/CD34+/CD117+/ CD13+/CD33+/CD56+
(No change) |
Clinical and laboratory criteria of 50 AML patients are shown in Table 1. LAIPs were observed in 43 cases (86.0%). Only one aberrant immunophenotype was identified in four cases (9.3%), but in the other 39 cases (90.7%), two to 12 aberrant immunophenotypes were identified (2 LAIPs, 8 cases; 3 LAIPs, 8 cases; 4 LAIPs, 4 cases; 5 LAIPs, 8 cases; 6 LAIPs, 5 cases; 7 LAIPs, 3 cases; 8 LAIPs, 1 case; 9 LAIPs, 1 case; and 12 LAIPs, 1 case). The most frequent LAIPs identified were in order of frequency; lack of antigen expression (73 times), followed by asynchronous antigen expression of progenitor cell markers and differentiation markers (58 times), and cross-lineage antigen expression (41 times). The percentage of AML cells carrying specific LAIPs was assessed in each case and ranged from 7.0% to 99.6% (median, 64.9%) [Table 4].
The most common LAIPs identified were CD117+/CD34- in 21/50 (42.0%), CD34+/CD7+ in 12/50 (24.0%), and CD33+/HLA-DR- in 12/50 (24.0%). Cross-lineage antigen expression was detected 41 times. The most frequent lymphoid antigen detected was CD7 in 12 (24.0%) of 50 cases, followed by CD19 in eight (16.0%) cases, CD2 in five (10.0%) cases, and CD4 in one (2.0%) case. At least one myeloid marker was absent 21 times, with CD13 the most frequent marker absent in 6/50 (12.0%) of cases.
Percentage of LAIPs positive cells within control BM samples were determined, and the median percentage for each LAIP was calculated, ranging from 0.00% to 0.02% [Table 4]. LAIPs in control BM samples showing median percentage > 0.1% as CD34+/CD117- (0.34%) and CD117+/CD34- (0.31%) are considered to have poor specificity.
Seven (14.0%) refractory cases were examined, 2/7 showed no LAIPs neither at initial diagnosis nor at persistent disease. The remaining cases 5/7 showed the presence of the same LAIPs at both initial diagnosis and persistent disease. Two (4.0%) relapsed cases were examined, 1/2 showed no LAIPs neither at initial diagnosis nor at relapse, while the other showed acquisition of new LAIPs after relapse [Table 5].
Discussion
The monitoring of MRD has become increasingly important to guide therapy in patients with AML. It plays an important role in the identification of patients with a high risk of relapse. MRD detection is now used in some of the recent protocols to guide patient-tailored therapy in AML.21 However, the ability of MRD by MFC to predict relapse is still unsatisfactory.
The main challenge depends on the correct choice of the number and types of LAIPs.13 In this study, we used an eight-color MFC and an extensive panel of MoAb, blasts were identified with the help of CD45/SSC gating strategy depending on dim expression of the CD45 antigen. This method was previously validated with LAIPs detected in 86.0% of cases.6
More than one LAIP was detected in 90.7% of cases; one case showed up to 12 LAIPs. The detection of several LAIPs in the same case can help in cases of immunophenotypic switch during treatment,22,23 and further improves the use of LAIPs strategy in MRD by MFC. Previously published articles used eight-color MFC on normal BM with the aim of subdividing LAIPs in categories of specificity into strong LAIPs (≤ 0.01%), good (> 0.01% but < 0.1%), and weak (> 0.1%).13
Our results were similar to Cui et al,5 in the order of LAIPs frequency and confirmed the findings of Rossi et al,13 and Al-Mawali et al,6 that strong LAIPs were obtained by a combination of CD2, CD4, CD56 with either CD34 or CD117, in contrast to CD19 which has to be combined with CD117 to be classified as ‘strong LAIPs’. Lack of antigen expression, although was the most frequent group in our study (73 times), yet most of them included CD117+/CD34- (42.0% of cases) and CD33+/HLA-DR- (24.0% of cases), which are considered to be ‘weak LAIPs’ due to their low specificity.
CD33-based aberrancies unassociated with immature markers such as CD34 or CD117 were excluded from our study as they did not have a clear role as LAIPs.13
Further investigation on a larger number of cases and correlation with cytogenetic/molecular data should be done to conclude whether there is an alteration in LAIPs in refractory/relapsed cases than those detected at diagnosis.
Eight-color MFC helped in better population identification and was found to be extremely useful in small specimens with fewer cells. Also, fewer tubes were used; therefore, saving reagents and instrument time. The good choice of LAIPs depends on their specificity rather than their frequency. Thus, it is important that the patients selected for MRD monitoring should have more than two LAIPs; at least one ‘strong’, two ‘good’ or three ‘weak’ LAIPs as stated by Rossi et al,13 to avoid false negative results.
Conclusion
The good choice of LAIPs depends on their specificity rather than their frequency. Therefore, this study’s results can help increase the sensitivity of LAIPs strategy in MRD using MFC in AML patients, which is considered an important post-diagnosis parameter associated with prognosis and clinical outcome.
Disclosure
The authors declared no conflicts of interest. No funding was received for this study.
references
- 1. Döhner H, Weisdorf DJ, Bloomfield CD. Acute myeloid leukemia. N Engl J Med 2015 Sep;373(12):1136-1152.
- 2. Sui JN, Chen QS, Zhang YX, Sheng Y, Wu J, Li JM, et al. Identifying leukemia-associated immunophenotype-based individualized minimal residual disease in acute myeloid leukemia and its prognostic significance. Am J Hematol 2019 May;94(5):528-538.
- 3. Döhner H, Estey E, Grimwade D, Amadori S, Appelbaum FR, Büchner T, et al. Diagnosis and management of AML in adults: 2017 ELN recommendations from an international expert panel. Blood 2017 Jan;129(4):424-447.
- 4. Venditti A, Buccisano F, Del Poeta G, Maurillo L, Tamburini A, Cox C, et al. Level of minimal residual disease after consolidation therapy predicts outcome in acute myeloid leukemia. Blood 2000 Dec;96(12):3948-3952.
- 5. Cui W, Zhang D, Cunningham MT, Tilzer L. Leukemia-associated aberrant immunophenotype in patients with acute myeloid leukemia: changes at refractory disease or first relapse and clinicopathological findings. Int J Lab Hematol 2014 Dec;36(6):636-649.
- 6. Al-Mawali A, Gillis D, Hissaria P, Lewis I. Incidence, sensitivity, and specificity of leukemia-associated phenotypes in acute myeloid leukemia using specific five-color multiparameter flow cytometry. Am J Clin Pathol 2008 Jun;129(6):934-945.
- 7. Chatterjee T, Mallhi RS, Venkatesan S. Minimal residual disease detection using flow cytometry: applications in acute leukemia. Med J Armed Forces India 2016 Apr;72(2):152-156.
- 8. Kern W, Danhauser-Riedl S, Ratei R, Schnittger S, Schoch C, Kolb HJ, et al. Detection of minimal residual disease in unselected patients with acute myeloid leukemia using multiparameter flow cytometry for definition of leukemia-associated immunophenotypes and determination of their frequencies in normal bone marrow. Haematologica 2003 Jun;88(6):646-653.
- 9. Kern W, Voskova D, Schoch C, Hiddemann W, Schnittger S, Haferlach T. Determination of relapse risk based on assessment of minimal residual disease during complete remission by multiparameter flow cytometry in unselected patients with acute myeloid leukemia. Blood 2004 Nov;104(10):3078-3085.
- 10. Schuurhuis GJ, Heuser M, Freeman S, Béné MC, Buccisano F, Cloos J, et al. Minimal/measurable residual disease in AML: a consensus document from the European LeukemiaNet MRD working party. Blood 2018 Mar;131(12):1275-1291.
- 11. Rossi G, Minervini MM, Melillo L, di Nardo F, de Waure C, Scalzulli PR, et al. Predictive role of minimal residual disease and log clearance in acute myeloid leukemia: a comparison between multiparameter flow cytometry and Wilm’s tumor 1 levels. Ann Hematol 2014 Jul;93(7):1149-1157.
- 12. Olaru D, Campos L, Flandrin P, Nadal N, Duval A, Chautard S, et al. Multiparametric analysis of normal and postchemotherapy bone marrow: implication for the detection of leukemia-associated immunophenotypes. Cytometry B Clin Cytom 2008 Jan;74(1):17-24.
- 13. Rossi G, Giambra V, Minervini MM, De Waure C, Mancinelli S, Ciavarella M, et al. Leukemia-associated immunophenotypes subdivided in “categories of specificity” improve the sensitivity of minimal residual disease in predicting relapse in acute myeloid leukemia. Cytometry B Clin Cytom 2020 May;98(3):216-225.
- 14. Langebrake C, Brinkmann I, Teigler-Schlegel A, Creutzig U, Griesinger F, Puhlmann U, et al. Immunophenotypic differences between diagnosis and relapse in childhood AML: implications for MRD monitoring. Cytometry B Clin Cytom 2005 Jan;63(1):1-9.
- 15. Voskova D, Schoch C, Schnittger S, Hiddemann W, Haferlach T, Kern W. Stability of leukemia-associated aberrant immunophenotypes in patients with acute myeloid leukemia between diagnosis and relapse: comparison with cytomorphologic, cytogenetic, and molecular genetic findings. Cytometry B Clin Cytom 2004 Nov;62(1):25-38.
- 16. Li X, Du W, Liu W, Li X, Li H, HUANG SA. Comprehensive flow cytometry phenotype in acute leukemia at diagnosis and at relapse. APMIS 2010;118(5):353-359.
- 17. Swerdlow SH. Mantle cell lymphoma. In: Swerdlow SH, Campo E, Harris NL, Jaffe ES, Pileri SA, Stein H, et al, editors. WHO classification of tumours of haematopoietic and lymphoid tissues. 4th ed. Lyon: IARC; 2017. p. 285-90.
- 18. Aalbers AM, van den Heuvel-Eibrink MM, Baumann I, Dworzak M, Hasle H, Locatelli F, et al. Bone marrow immunophenotyping by flow cytometry in refractory cytopenia of childhood. Haematologica 2015 Mar;100(3):315-323.
- 19. Kalina T, Flores-Montero J, van der Velden VH, Martin-Ayuso M, Böttcher S, Ritgen M, et al; EuroFlow Consortium (EU-FP6, LSHB-CT-2006-018708). EuroFlow standardization of flow cytometer instrument settings and immunophenotyping protocols. Leukemia 2012 Sep;26(9):1986-2010.
- 3. Cloos J, Harris JR, Janssen JJ, Kelder A, Huang F, Sijm G, et al. Comprehensive protocol to sample and process bone marrow for measuring measurable residual disease and leukemic stem cells in acute myeloid leukemia. J Vis Exp 2018 Mar;(133):56386.
- Lambert J, Lambert J, Nibourel O, Pautas C, Hayette S, Cayuela JM, et al. MRD assessed by WT1 and NPM1 transcript levels identifies distinct outcomes in AML patients and is influenced by gemtuzumab ozogamicin. Oncotarget 2014 Aug;5(15):6280-6288.
- 21. Bresters D, Reus AC, Veerman AJ, van Wering ER, van der Does-van den Berg A, Kaspers GJ. Congenital leukaemia: the Dutch experience and review of the literature. Br J Haematol 2002 Jun;117(3):513-524.
- 22. Plata E, Choremi-Papadopoulou H, Viglis V, Yataganas X. Flow-cytometric detection of minimal residual disease with atypical antigen combinations in patients with de novo acute myeloid leukemia. Ann Hematol 2000 Oct;79(10):543-546.