Urinary tract infections (UTIs) are one of the most prevalent infectious diseases in both hospital and community settings and can lead to a high rate of morbidity and mortality.1,2 Various pathogens are responsible for causing UTIs, of which Escherichia coli account for the majority of infections.3
Among the array of antibiotics commonly used for the treatment of UTIs, β-lactams are the most extensively used agents.1 However, the emergence of multiple-drug resistant (MDR) strains, particularly extended-spectrum beta-lactamases (ESBLs) producing strains, has become a global healthcare concern.4–8 Recently, the trend of ESBL-producing E. coli strains has increased worldwide, including in Iran.9,10 ESBLs are enzymes capable of hydrolyzing penicillins, broad-spectrum cephalosporins, and monobactams.11 The specific ESBL-producing strains have different genetic characteristics, which may cause specific pathogenesis characteristics.4,12
The antibiotic resistance determinants can normally transfer among bacterial strains by different horizontal gene transfer mechanisms, including integrons.13 These elements are responsible for the integration and dissemination of resistance genes among the bacteria. To date, several classes of integrons have been described based on the amino acid sequences of their integrase genes (intI).13 Of them, class 1 and 2 integrons are the most prevalent classes of MDR gram-negative bacteria associated with antibiotic treatment failure.14
Successful colonization and invasion of E. coli into host uroepithelial cells and bladder tissues is mostly dependent on fimbrial adhesions.15 Among adhesions of uropathogenic E. coli (UPEC), fimH protein is a major virulence determinant, which plays an important role in host-pathogen interactions and biofilm formation.15 These features made fimH a worthy therapeutic target for the development of new methods for the diagnosis and prevention of UTIs.
Awareness of the trends of antibiotic resistance and mechanisms of resistance is a rational way to overcome the risk of drug resistance and treatment failure. Thus, we investigated the antimicrobial resistance pattern and presence of integrons and fimH determinants among UPEC isolates obtained from hospitalized Iranian patients.
Methods
This cross-sectional study was performed using 121 non-duplicated E. coli isolates obtained from hospitalized patients with symptomatic UTIs at Nemazee teaching hospital in Shiraz, Southwestern Iran from November 2016 to May 2017. This study was in accordance with the declaration of Helsinki and approved by the Ethics Committee of Shiraz University of Medical Sciences.
The isolation and identification of E. coli strains were performed by standard microbiological tests and confirmed by API 20E strip (API-bioMérieux, France). The confirmed isolates were stored at -80 °C for long-term preservation.
Antibiotic susceptibility of all isolates to amikacin (30 µg), ampicillin (10 µg), ceftazidime (30 µg), imipenem (10 µg), tetracycline (30 µg), levofloxacin (5 µg), ciprofloxacin (5 µg), nalidixic acid (30 µg), nitrofurantoin (300 µg), co-trimoxazole (25 µg), and gentamicin (10 µg) (Mast Co., UK) was carried out on Muller- Hinton agar (Merck, Germany) using the disk diffusion method as recommended by the Clinical and Laboratory Standards Institute (CLSI).16 E. coli ATCC 25922 was used as a quality control strain for antibacterial susceptibility testing. MDR was estimated according to previously described definitions (i.e., non-susceptible to ≥ 1 agent in ≥ 3 different antimicrobial categories).17
All isolates were tested for ESBL production, using the double-disk synergy test using ceftazidime (30 µg) and ceftazidime-clavulanic acid (30/10 µg) disks as described by CLSI guidelines.16 E. coli ATCC 25922 and Klebsiella pneumoniae ATCC 700603 were used as negative and positive control strains, respectively.
The template DNA used was extracted from freshly grown colonies by the boiling method as described previously.18 Simplex polymerase chain reaction (PCR) was used to determine the presence of classes 1–3 integrons by detection of integrase genes intI1, intI2 and intI3, and fimH (type-1 fimbriae), using the previously described primers [Table 1].19,20 PCR amplifications for the studied genes were carried out in the following condition, initial denaturation at 95 °C for 5 minutes, followed by 30 cycles of denaturation at 95 °C for 60 seconds, annealing for 45 seconds (temperature dependent on primer sequences), extension at 72 °C for 50 seconds, and final extension at 72 °C for 5 minutes. PCR amplifications for the studied genes was carried out on a T100™ thermal cycler (Bio-Rad, Hercules, CA, USA). The amplifications were separated on 1.5% agarose gel prepared in 1X TAE (Tris/Acetate/EDTA) buffer and visualized using ultraviolet light after staining with safe stain load dye (CinnaGen Co., Iran) [Figure 1]. The purified PCR products of the amplified intI1 and intI2 genes were submitted for sequencing (Bioneer Co., South Korea), and the sequences were compared using online BLAST software. For fimH gene, E. coli ATCC 25922 was used as a positive control strain.
The analysis was performed using SPSS Statistics (IBM Corp. Released 2012. IBM SPSS Statistics for Windows, Version 21.0. Armonk, NY: IBM Corp). The results are presented as descriptive statistics in terms of relative frequency. Values are expressed as the percentages of the group (categorical variables). Chi-square or Fisher’s exact tests were used to determine the significance of the differences. A difference was considered statistically significant if the p-value was < 0.050.
Table 1: List of primers used.
IntI1-Forward |
GGTCAAGGATCTGGATTTCG |
intI1 |
483 |
19 |
IntI1-Reverse |
ACATGCGTGTAAATCATCGTC |
IntI2-Forward |
CACGGATATGCGACAAAAAGGT |
intI2 |
789 |
19 |
IntI2-Reverse |
GTAGCAAACGAGTGACGAAATG |
IntI3-Forward |
AGTGGGTGGCGAATGAGTG |
intI3 |
600 |
19 |
IntI3-Reverse |
TGTTCTTGTATCGGCAGGTG |
FimH-Forward |
TGCAGAACGGATAAGCCGTGG |
Table 2: The results of antibiotic susceptibility pattern of Escherichia coli isolates.
Penicillins |
Ampicillin |
11 |
9.1 |
11 |
15.7 |
0 |
0.0 |
< 0.001 |
Cephalosporins |
Ceftazidime |
40 |
33.1 |
39 |
55.7 |
1 |
2.0 |
< 0.001 |
Tetracyclines |
Tetracycline |
39 |
32.2 |
28 |
40.0 |
11 |
21.6 |
0.032 |
Quinolones |
Nalidixic acid |
23 |
19.0 |
17 |
24.3 |
6 |
11.8 |
0.083 |
Ciprofloxacin |
50 |
41.3 |
37 |
52.9 |
13 |
25.5 |
0.003 |
Levofloxacin |
60 |
49.6 |
43 |
61.4 |
17 |
33.3 |
0.002 |
Sulfonamides |
Co-trimoxazole |
30 |
24.8 |
20 |
28.6 |
10 |
19.6 |
0.260 |
Aminoglycosides |
Gentamicin |
78 |
64.5 |
53 |
75.7 |
25 |
49.0 |
0.002 |
Amikacin |
86 |
71.1 |
51 |
72.9 |
35 |
68.6 |
0.610 |
Nitrofurans |
Nitrofurantoin |
93 |
76.9 |
56 |
80.0 |
37 |
72.5 |
0.340 |
ESBL: extended-spectrum beta-lactamase.
Table 3: Antibiotic susceptibility pattern of the studied Escherichia coli isolates according to integron class 1 positivity.
Penicillins |
Ampicillin |
4 |
8.2 |
7 |
9.7 |
0.770 |
Cephalosporins |
Ceftazidime |
15 |
30.6 |
25 |
34.7 |
0.640 |
Tetracyclines |
Tetracycline |
19 |
38.8 |
20 |
27.8 |
0.200 |
Quinolones |
Nalidixic acid |
11 |
22.4 |
12 |
16.7 |
0.430 |
Ciprofloxacin |
21 |
42.9 |
29 |
40.3 |
0.780 |
Levofloxacin |
27 |
55.1 |
33 |
45.8 |
0.320 |
Sulfonamides |
Co-trimoxazole |
17 |
34.7 |
13 |
18.1 |
0.037 |
Aminoglycosides |
Gentamicin |
32 |
63.5 |
46 |
63.9 |
0.870 |
Amikacin |
33 |
67.3 |
53 |
73.6 |
0.460 |
Nitrofurans |
Nitrofurantoin |
42 |
85.7 |
51 |
70.8 |
0.057 |
Results
Of 121 E. coli isolates included in our study, 74 (61.2%) and 47 (38.8%) isolates were obtained from females and males patients, respectively. The median age of the patients was 52.1±27.8 years, ranging from 1 month to 100 years old. The most frequent source of bacterial isolation was from intensive care units (59.5%) followed by internal wards (16.5%), neonatal intensive care units (14.9%), surgery wards (6.6%), and the transplantation ward (2.5%).
Imipenem (77.7%) and nitrofurantoin (76.9%) were the most effective in vitro antibiotics for E. coli isolates. Ampicillin (9.1%) and nalidixic acid (19.0%) showed the lowest level of susceptibility. The full results of antibiotic susceptibility pattern for UPEC isolates are shown in Table 2.
The rate of ESBL-producing isolates was 42.1% (51/121). There was a significant association between production of ESBLs and higher antibiotic resistance in the tested isolates [Table 2]. The most effective antibiotic against ESBL-producing isolates was nitrofurantoin followed by amikacin and imipenem. In total, 98.0% of the ESBL-producing isolates and 87.1% of non-ESBL-producing isolates were MDR (p = 0.040).
Seventy-two (59.5%) isolates were positive for int1 gene and harboring class 1 integron; nine (7.4%) isolates were positive for intI2 genes and harboring class 2 integron, and seven (5.8%) isolates contained both genes. Class 3 integron was not detected in any of the isolates by the absence of intI3 gene. Integron class 1 was significantly associated with lower susceptibility to sulfonamides (p < 0.050) [Table 3]. Moreover, there was no significant association between ESBLs production and integron 1 or 2 positivity. The incidence of integron class 1 and 2 among MDR isolates was 95.8% and 100%, respectively. These rates in non-MDR isolates were 85.7% for class 1 integron and 91.1% for class 2 integron (p > 0.050). Type 1 fimbrial adhesin by detection of fimH gene was positive in 98.3% of UPEC isolates.
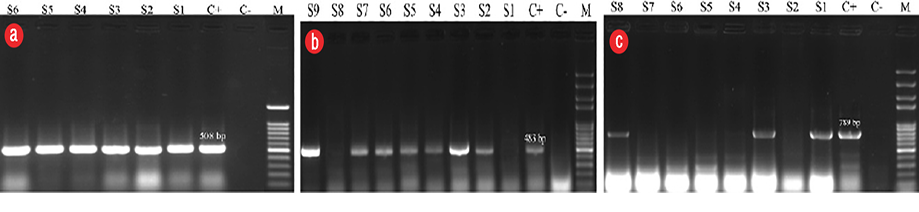
Figure 1: Agarose gel electrophoresis of polymerase chain reaction (PCR) products for (a) fimH gene,
(b) intI1gene and (c) intI2 gene. Lane M: 100bp DNA size marker, column C-: negative control, C+: positive control, S: results of clinical samples.
Discussion
Nosocomial infections caused by drug-resistant E. coli spread rapidly in the world and can be associated with higher rates of morbidity and mortality in vulnerable populations, such as neonates, pregnant women, or immunocompromised patients.21 To reduce the risk of complications associated with hospital-acquired UPEC infections and overcome the dissemination of drug-resistant strains, we strongly recommend periodic surveillance to identify the ESBL-producing strains to optimize available infection control policies.22
We report the antibiotic resistance and prevalence of ESBL-producing UPEC isolates in Southwestern Iran. The prevalence of ESBL-producing UPEC isolates was 42.1%, which in spite of the great discrepancy is consistent with the median values (24% to 72.9%) reported in previous studies from Iran.23–30 The differences globally in the prevalence of ESBL-producing UPEC isolates can be due to differences in geographical regions, infection control policies, the origin of infection, and sample size.
ESBLs are plasmid-mediated β-lactamases that are capable of hydrolyzing β-lactams, except for carbapenems and cephamycins.11,31 In this regard, the majority of our ESBL-producing isolates were resistant to penicillins and cephalosporins. Moreover, in accordance with previous reports,23,24,31,32 our ESBL-producing isolates showed significantly higher resistance rates to most of the tested agents. Similar to our findings, several authors have introduced carbapenem, amikacin, and nitrofurantoin as a treatment option for UTIs caused by ESBL-producing E. coli strains.29,32,33
Class 1 and 2 integron-integrase genes were found in 59.5% and 7.4% of isolates, respectively. Numerous studies reported the prevalence of integrons among clinical isolates of E. coli from Iran and other parts of the world. Generally, those studies reported a higher incidence of class 1 integron34–37; however, there were some variations according to geographical distribution and source of infections. One report from Yasuj, southwest of Iran showed class 1 and 2 integrons in 52%, and 2.5% of UPEC isolates, respectively.34 In the northern part of the country, only 22% of UPEC isolates contained the class 1 integron gene.38 In Northwest Iran, the prevalence of class 1 and 2 integrons was 22.05% and 5.08% of MDR UPEC strains, respectively.39 The highest rate of integrons carrying UPEC isolates was reported from the west of Iran with 87% for class 1, and 7% for class 2.37 It seems that the occurrence of integrons in the community and hospitals can be different. Another study conducted in our region (Fars province) showed the prevalence of class 1 and 2 integrons in community-acquired UPEC isolates to be 6.25% and 10.41%, respectively.40
In our results, in agreement with previous studies, almost all of the UPEC isolates (98.3%) carried the fimH gene.41–44 Our observations suggest the potential of type 1 fimbrial adhesin as promising candidates for the development of new therapeutic strategies to prevent complications related to UPEC colonization.45
Conclusion
The remarkable rate of MDR and ESBL-producing UPEC isolates accompanied with the presence of integrons suggests the necessity of restricted infection control policies to prevent further dissemination of resistant strains. Several locally available antibiotics showed promising effects against our MDR isolates. Due to the continuous evolution of pathogens in hospital environments continuous updating of local data, such as this study, provide experimental evidence to improve the outcome of nosocomial infections.
Disclosure
The authors declared no conflicts of interest. This study was supported by Student Research Committee, Shiraz University of Medical Sciences, grant No. 95-11558.
Acknowledgements
We thank all personnel at Microbiology laboratory for their technical assistance. The authors wish to thank Dr. Nasrin Shokrpour at the Research Consolation Center at Shiraz University of Medical Sciences for her invaluable assistance in editing this manuscript.
references
- 1. Flores-Mireles AL, Walker JN, Caparon M, Hultgren SJ. Urinary tract infections: epidemiology, mechanisms of infection and treatment options. Nat Rev Microbiol 2015 May;13(5):269-284.
- 2. Zorgani A, Almagatef A, Sufya N, Bashein A, Tubbal A. Detection of CTX-M-15 among uropathogenic Escherichia coli isolated from five major hospitals in Tripoli, Libya. Oman Med J 2017 Jul;32(4):322-327.
- 3. Ronald A. The etiology of urinary tract infection: traditional and emerging pathogens. Dis Mon 2003 Feb;49(2):71-82.
- 4. Shaikh S, Fatima J, Shakil S, Rizvi SM, Kamal MA. Antibiotic resistance and extended spectrum beta-lactamases: Types, epidemiology and treatment. Saudi J Biol Sci 2015 Jan;22(1):90-101.
- 5. Hashemi SH, Hashemi N, Esna-Ashari F, Taher A, Dehghan A. Clinical features and antimicrobial resistance of bacterial agents of ventilator-associated tracheobronchitis in Hamedan, Iran. Oman Med J 2017 Sep;32(5):403-408.
- 6. Heidari H, Hasanpour S, Ebrahim-Saraie HS, Motamedifar M. High incidence of virulence factors among clinical enterococcus faecalis isolates in Southwestern Iran. Infect Chemother 2017 Mar;49(1):51-56.
- 7. Houri H, Kazemian H, Sedigh Ebrahim-Saraie H, Taji A, Tayebi Z, Heidari H. Linezolid activity against clinical Gram-positive cocci with advanced antimicrobial drug resistance in Iran. J Glob Antimicrob Resist 2017 Sep;10:200-203.
- 8. Motamedifar M, Ebrahim-Saraie HS, Abadi AR, Moghadam MN. First outcome of MDR-TB among Co-Infected HIV/TB patients from South-West Iran. Tuberc Respir Dis (Seoul) 2015 Jul;78(3):253-257.
- 9. Pourakbari B, Ferdosian F, Mahmoudi S, Teymuri M, Sabouni F, Heydari H, et al. Increase resistant rates and ESBL production between E. coli isolates causing urinary tract infection in young patients from Iran. Braz J Microbiol 2012 Apr;43(2):766-769.
- 10. Kazemian H, Heidari H, Ghanavati R, Mohebi R, Ghafourian S, Shavalipour A, et al. Characterization of antimicrobial resistance pattern and molecular analysis among extended spectrum β-lactamase-producing Escherichia coli. Pharm Sci 2016;22(4):279-284.
- 11. Drawz SM, Bonomo RA. Three decades of beta-lactamase inhibitors. Clin Microbiol Rev 2010 Jan;23(1):160-201.
- 12. Paterson DL, Bonomo RA. Extended-spectrum beta-lactamases: a clinical update. Clin Microbiol Rev 2005 Oct;18(4):657-686.
- 13. Domingues S, da Silva GJ, Nielsen KM. Integrons: Vehicles and pathways for horizontal dissemination in bacteria. Mob Genet Elements 2012 Sep;2(5):211-223.
- 14. Deng Y, Bao X, Ji L, Chen L, Liu J, Miao J, et al. Resistance integrons: class 1, 2 and 3 integrons. Ann Clin Microbiol Antimicrob 2015;14:45.
- 15. Mulvey MA. Adhesion and entry of uropathogenic Escherichia coli. Cell Microbiol 2002 May;4(5):257-271.
- 16. Wayne PA. Performance standards for antimicrobial susceptibility testing. Clinical and Laboratory Standards Institute (CLSI) 2015;25th Informational Supplement.(M100-S25).
- 17. Magiorakos AP, Srinivasan A, Carey RB, Carmeli Y, Falagas ME, Giske CG, et al. Multidrug-resistant, extensively drug-resistant and pandrug-resistant bacteria: an international expert proposal for interim standard definitions for acquired resistance. Clin Microbiol Infect 2012 Mar;18(3):268-281.
- 18. Heidari H, Emaneini M, Dabiri H, Jabalameli F. Virulence factors, antimicrobial resistance pattern and molecular analysis of Enterococcal strains isolated from burn patients. Microb Pathog 2016 Jan;90:93-97.
- 19. Machado E, Cantón R, Baquero F, Galán JC, Rollán A, Peixe L, et al. Integron content of extended-spectrum-beta-lactamase-producing Escherichia coli strains over 12 years in a single hospital in Madrid, Spain. Antimicrob Agents Chemother 2005 May;49(5):1823-1829.
- 20. Chapman TA, Wu XY, Barchia I, Bettelheim KA, Driesen S, Trott D, et al. Comparison of virulence gene profiles of Escherichia coli strains isolated from healthy and diarrheic swine. Appl Environ Microbiol 2006 Jul;72(7):4782-4795.
- 21. Friedman ND, Temkin E, Carmeli Y. The negative impact of antibiotic resistance. Clin Microbiol Infect 2016 May;22(5):416-422.
- 22. Harris AD, McGregor JC, Johnson JA, Strauss SM, Moore AC, Standiford HC, et al. Risk factors for colonization with extended-spectrum beta-lactamase-producing bacteria and intensive care unit admission. Emerg Infect Dis 2007 Aug;13(8):1144-1149.
- 23. Mehdipour Moghaddam MJ, Mirbagheri AA, Salehi Z, Habibzade SM. Prevalence of class 1 integrons and extended spectrum Beta Lactamases among multi-drug resistant Escherichia coli isolates from North of Iran. Iran Biomed J 2015;19(4):233-239.
- 24. Damavandi MS, Gholipour A, Latif Pour M. Prevalence of class D carbapenemases among extended-spectrum β-Lactamases producing Escherichia coli isolates from educational hospitals in Shahrekord. J Clin Diagn Res 2016 May;10(5):DC01-DC05.
- 25. Sedighi I, Arabestani MR, Rahimbakhsh A, Karimitabar Z, Alikhani MY. Dissemination of extended-spectrum β-Lactamases and quinolone resistance genes among clinical isolates of uropathogenic Escherichia coli in children. Jundishapur J Microbiol 2015 Jul;8(7):e19184.
- 26. Harifi Mood E, Meshkat Z, Izadi N, Rezaei M, Amel Jamehdar S, Naderi Nasab M. Prevalence of quinolone resistance genes among extended-spectrum β-Lactamase-producing Escherichia coli in Mashhad, Iran. Jundishapur J Microbiol 2015 Dec;8(12):e16217.
- 27. Moosavian M, Ahmadkhosravy N. Survey of CTX-M gene frequency in extended-spectrum Beta-Lactamase-Producing Enterobacteriaceae isolates using the combination disk and PCR methods in Ahvaz, Iran. Jundishapur J Microbiol 2016 Sep;9(11):e40423.
- 28. Tayebi Z, Heidari H, Kazemian H, Ghafoori SM, Boroumandi S, Houri H. Comparison of quinolone and beta-lactam resistance among Escherichia coli strains isolated from urinary tract infections. Infez Med 2016 Dec;24(4):326-330.
- 29. Pouladfar G, Basiratnia M, Anvarinejad M, Abbasi P, Amirmoezi F, Zare S. The antibiotic susceptibility patterns of uropathogens among children with urinary tract infection in Shiraz. Medicine (Baltimore) 2017 Sep;96(37):e7834.
- 30. Zamani K, Emami A, Bazargani A, Moattari A. Phenotypic and molecular characterization of CTX-M extended-spectrum beta-lactamase-producing Escherichia coli isolates in Shiraz, Iran. Rev Soc Bras Med Trop 2015 Jul-Aug;48(4):479-482.
- 31. Haghighatpanah M, Mozaffari Nejad AS, Mojtahedi A, Amirmozafari N, Zeighami H. Detection of extended-spectrum β-lactamase (ESBL) and plasmid-borne blaCTX-M and blaTEMgenes among clinical strains of Escherichia coli isolated from patients in the north of Iran. J Glob Antimicrob Resist 2016 Dec;7:110-113.
- 32. Zeighami H, Haghi F, Hajiahmadi F. Molecular characterization of integrons in clinical isolates of betalactamase-producing Escherichia coli and Klebsiella pneumoniae in Iran. J Chemother 2015 Jun;27(3):145-151.
- 33. Tabar MM, Mirkalantari S, Amoli RI. Detection of ctx-M gene in ESBL-producing E. coli strains isolated from urinary tract infection in Semnan, Iran. Electron Physician 2016 Jul;8(7):2686-2690.
- 34. Khoramrooz SS, Sharifi A, Yazdanpanah M, Malek Hosseini SA, Emaneini M, Gharibpour F, et al. High frequency of class 1 integrons in Escherichia coli isolated from patients with urinary tract infections in Yasuj, Iran. Iran Red Crescent Med J 2016 Jan;18(1):e26399.
- 35. Solberg OD, Ajiboye RM, Riley LW. Origin of class 1 and 2 integrons and gene cassettes in a population-based sample of uropathogenic Escherichia coli. J Clin Microbiol 2006 Apr;44(4):1347-1351.
- 36. Gündoğdu A, Long YB, Vollmerhausen TL, Katouli M. Antimicrobial resistance and distribution of sul genes and integron-associated intI genes among uropathogenic Escherichia coli in Queensland, Australia. J Med Microbiol 2011 Nov;60(Pt 11):1633-1642.
- 37. Zeighami H, Haghi F, Masumian N, Hemmati F, Samei A, Naderi G. Distribution of integrons and gene cassettes among uropathogenic and diarrheagenic Escherichia coli isolates in Iran. Microb Drug Resist 2015 Aug;21(4):435-440.
- 38. Ahangarkani F, Rajabnia R, Shahandashti EF, Bagheri M, Ramez M. Frequency of class 1 integron in Escherichia coli strains isolated from patients with urinary tract infections in north of iran. Mater Sociomed 2015 Feb;27(1):10-12.
- 39. Rezaee MA, Sheikhalizadeh V, Hasani A. Detection of integrons among multi-drug resistant (MDR) Escherichia coli strains isolated from clinical specimens in northern west of Iran. Braz J Microbiol 2011 Oct;42(4):1308-1313.
- 40. Farshad S, Japoni A, Hosseini M. Low distribution of integrons among multidrug resistant E. coli strains isolated from children with community-acquired urinary tract infections in Shiraz, Iran. Pol J Microbiol 2008;57(3):193-198.
- 41. Lee JH, Subhadra B, Son YJ, Kim DH, Park HS, Kim JM, et al. Phylogenetic group distributions, virulence factors and antimicrobial resistance properties of uropathogenic Escherichia coli strains isolated from patients with urinary tract infections in South Korea. Lett Appl Microbiol 2016 Jan;62(1):84-90.
- 42. Qin X, Hu F, Wu S, Ye X, Zhu D, Zhang Y, et al. Comparison of adhesin genes and antimicrobial susceptibilities between uropathogenic and intestinal commensal Escherichia coli strains. PLoS One 2013 Apr;8(4):e61169.
- 43. Rahdar M, Rashki A, Miri HR, Rashki Ghalehnoo M. Detection of pap, sfa, afa, foc, and fim Adhesin-Encoding Operons in Uropathogenic Escherichia coli Isolates Collected From Patients With Urinary Tract Infection. Jundishapur J Microbiol 2015 Aug;8(8):e22647.
- 44. Yun KW, Kim HY, Park HK, Kim W, Lim IS. Virulence factors of uropathogenic Escherichia coli of urinary tract infections and asymptomatic bacteriuria in children. J Microbiol Immunol Infect 2014 Dec;47(6):455-461.
- 45. Sivignon A, Bouckaert J, Bernard J, Gouin SG, Barnich N. The potential of FimH as a novel therapeutic target for the treatment of Crohn’s disease. Expert Opin Ther Targets 2017 Sep;21(9):837-847.