Augmenting the immune system and its response has now become part of modern medical practice, including preventive measures, optimization of wellness, and even self-medication for mild illness. The regulation of the immune system is essential for the optimization of the immune response. The mechanism of immunomodulation is highly complex because it requires activation, proliferation, and differentiation of immune cells such as macrophages, T-helper cells, and B-cells. Macrophages and lymphocytes can guard and activate cellular defense with the help of molecular detectors such as toll-like receptors.1,2 Specific antibodies are generated in response to antigens or toxins to initiate the destruction of foreign cells or organisms.
The immunomodulatory properties of plants are being studied with greater interest in recent years. This is due to growing awareness regarding the need to modulate the immune system to achieve the desired effects of infection prevention and treatment of cancer and autoimmune diseases. A medicinal plant with an immunomodulatory effect could provide alternative substances or compounds for this purpose, especially concerning host defense mechanism therapy.3–5
The compounds responsible for therapeutic effects are usually plant secondary metabolites. Plant-derived products such as polysaccharides, lectins, peptides, flavonoids, and tannins have been used to modulate the immune response or immune system in various in vitro models.3 Immunomodulators could affect the human system by regulating immune properties such as phagocytic activity, cytokines release, and apoptotic activity in cells. Using a plant extract as a supplement could enhance health and wellness, especially in people with immunity impairment.
Quercus infectoria is a popular medicinal plant traditionally used in postpartum care and the treatment of various ailments. The galls of Q. infectoria are used as ‘jamu’ (i.e., a health supplement).6 Pharmacologically, the galls of Q. infectoria have been documented to possess astringent, antibacterial, and antifungal properties in vitro.7-10 In addition, the gall extract can inhibit the function of macrophages or neutrophils and inhibit the release of inflammatory mediators.4 Tannin, constituting almost 50–70% of Q. infectoria galls extract, is thought to be an important compound responsible for antimicrobial activity.5,11,12
Previous studies on the antimicrobial properties of Q. infectoria gall extracts have largely focused on their efficacy against certain pathogenic bacteria and fungi.13-17 Improved knowledge of the impact of this bioactive compound on the immune system is necessary. Antioxidant activity in plant materials, including Q. infectoria gall extracts, generally originates from their polyphenolic content.18 There is a lack of experimental data on the potential benefits of the extract as an immunomodulator. If such benefits could be demonstrated, Q. infectoria gall extract could be used as an alternative immunomodulator or supplement to minimize the side effects of cytotoxic drugs. This study aimed to explore the immunomodulatory potential of Q. infectoria gall water extract in vitro.
Methods
Galls of Q. infectoria were purchased from a local herb shop in Kota Bharu, Kelantan, Malaysia. Identification of the gall was made based on physical appearance.19 Aqueous extract was prepared by immersing 100 g of Q. infectoria gall powder in 500 mL of sterile distilled water for 72 hours in a 50 °C water bath. The mixture was then prefiltered using a coffee filter and followed with filtration using Whatman filter paper No 1. The filtrates were concentrated under reduced pressure using a rotary evaporator. The resulting pellet was freeze-dried at -50 °C under vacuum until the pellet produced a fine crystal-like crude extract. The crude extract was stored in airtight jars at 4 °C until use.17 Then, 100 mg of crude extract powder was added to 4 mL of sterile distilled water. Serial dilution was performed in Roswell Park Memorial Institute (RPMI) 1640 medium to obtain concentrations of 64 µg/mL, 32 µg/mL, and 16 µg/mL. The extract was freshly prepared before use.
This study used a murine macrophage (J774A.1) cell line (ATCC, USA) maintained in a complete growth medium (RPMI 1640) [Addex Bio & Sigma, USA] containing 12% fetal bovine serum [Gibco, USA] and 1% penicillin/streptomycin/neomycin under standard culture conditions (37 °C, 95% humidified air, and 5% carbon dioxide (CO2)). Cell passage was performed when the cell density reached 70–80% confluence. Cells were detached using a cell scraper and then aspirated into a 15 mL centrifuge tube. The flask was rinsed twice with pre-warmed growth medium and aspirated again into the 15 mL centrifuge tube. The medium containing cells was centrifuged at 1500 rpm for 5 minutes. The suspension was discarded, and the pellet was re-suspended with 1 mL growth medium. The cell suspension was transferred into five new 25 cm2 flasks containing 5 mL growth medium. For harvesting, the cells were transferred into a 15 mL centrifuge tube by scraping the cells and pelleted by centrifugation at 1500 rpm for 5 minutes. Cell pellets were washed and resuspended in 200 µL of phosphate-buffered saline or stored at -80 oC until use. Cell number and viability were assessed microscopically using trypan blue exclusion.
The effect of Q. infectoria gall extract on murine macrophage cell viability was evaluated using a MTT 3-(4,5-dimethylthiazol-2-yl)-2,5-di-phenyl-tetrazolium bromide (MTT) assay. In brief, cells were placed into the wells of a 96-well plate (2 × 103 cell per well) and incubated at 37 °C in a CO2 incubator for 24 hours. The medium was discarded and the wells received Q. infectoria gall extract in growth medium (64, 32, and 16 µg/mL) while a control well received medium only. The plate was incubated for 24, 48, and 72 hours at 37 °C in a CO2 incubator followed by the addition of 10 µL of MTT (5 µg/mL) solution into the wells. The plate was further incubated for 2 to 4 hours. Thereafter, 100 µL of stop solution dimethyl sulfoxide (DMSO) was added to each well to dissolve the formazan particles formed in the live cells. The absorbance of each cell concentration (optical density, OD) at 570 nm was measured in a plate reader. The MTT solution (yellow) crystalized the viable cell and changed it to formazan. The color of the solution then changed to purple. All tests were performed in triplicate. The OD in untreated control cells was taken as 100% of viability. Cell viability was expressed as a percentage (%) compared to the control (absence of any extract).
A phagocytic assay was performed according to the manufacturer’s instructions. Cells (1 × 105 cells/mL) were seeded in six wells and incubated overnight, followed by treatment with 64 μg/mL, 32 μg/mL, and 16 μg/mL of gall extract, as well as 10 μg/mL phytohemagglutinin (PHA) as a positive control. Then, 100 µg/mL of latex beads-rabbit IgG-FITC solution (Cayman Chemical, USA) was added into each well and left for 72 hours at ambient temperature. The cells were harvested, transferred into a polystyrene tube and centrifuged at 1200 rpm at room temperature for 5 minutes. The supernatant was aspirated from each tube, and 500 mL of assay buffer was added to the cells. The tubes were centrifuged again and the supernatant was aspirated 2-3 times. The final solution was vortexed to ensure that all cells were suspended. Samples were analyzed immediately by flow cytometry.
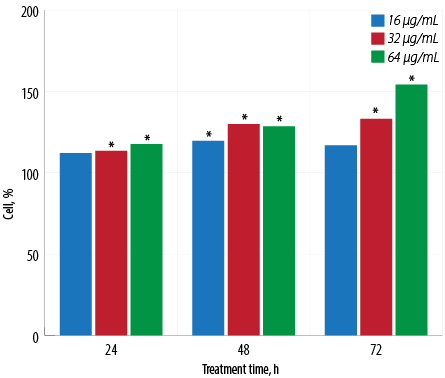
Figure 1: Proliferative activity of macrophages treated with different concentrations of aqueous Quercus infectoria gall extract after 24, 48, and 72 hours of incubation. The optical density in untreated control cells was taken as 100% of viability. Asterisk (*) indicates that statistically significant differences (p < 0.001) were observed between the percentage values of each concentration of extract-treated cells and untreated cells.
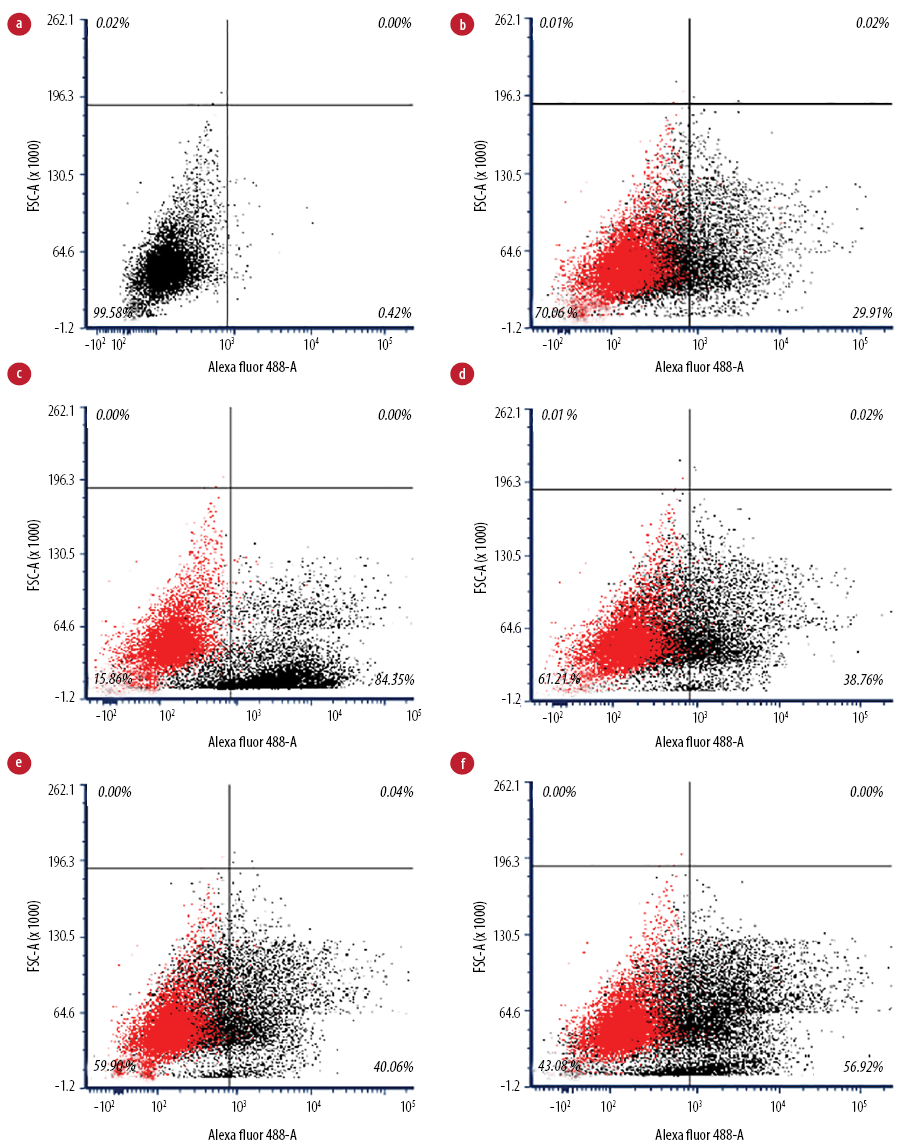
Figure 2: Flow cytometry analysis of IgG-coated bead phagocytic activity of macrophages. (a) Unstained macrophages, (b) untreated macrophages (negative control), (c) treated macrophages with phytohemagglutinin (positive control), (d) treated macrophages with 16 µg/mL, (e) treated macrophages with 32 µg/mL, and (f) treated macrophages with 64 µg/mL.
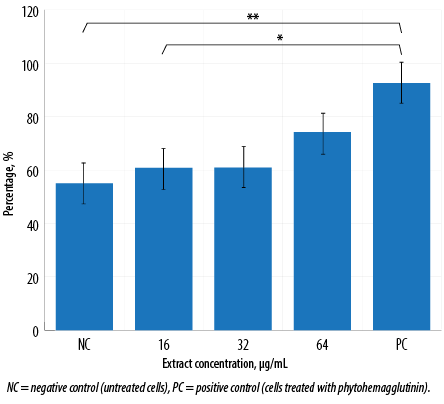
Figure 3: Phagocytic activity of macrophages treated with various concentrations of aqueous Quercus infectoria gall extract after 72 hours. The percentage of cells that phagocytose beads is indicated. This graph was tabulated from flow cytometry analysis. Asterisks indicate significant differences (p < 0.001) between PC and extract-treated cells at 16 µg/mL (*) and between PC and NC (**).
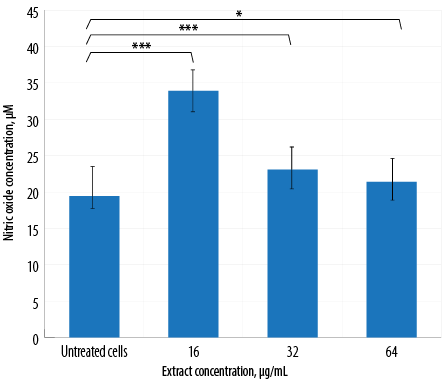
Figure 4: Nitric oxide production by macrophages treated with different concentrations of aqueous Quercus infectoria galls extract. The differences between the untreated and extract-treated cells were statistically significant (*** p < 0. 001; * p < 0.010).
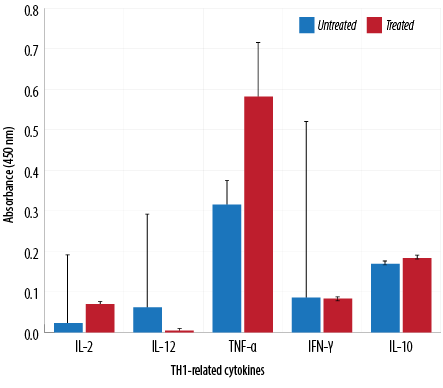
Figure 5: TH1-related cytokine analysis of macrophages treated with aqueous Quercus infectoria gall extract (64 µg/mL) in comparison to untreated macrophages.
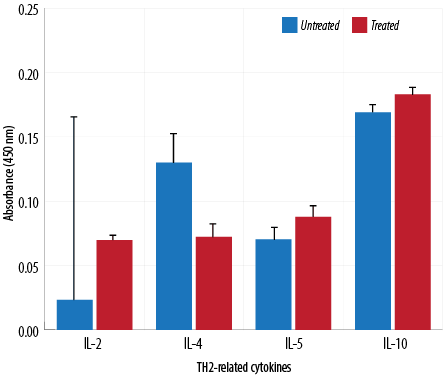
Figure 6: TH2-related cytokine analysis of macrophages treated with aqueous Quercus infectoria gall extract (64 µg/mL) in comparison to untreated macrophages.
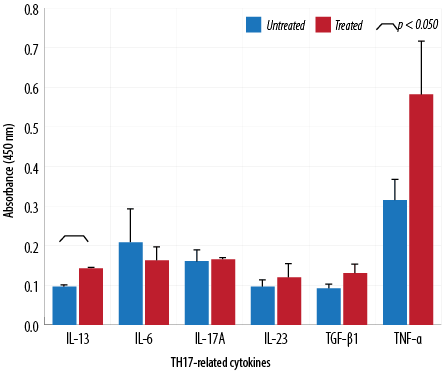
Figure 7: Cytokine analysis of macrophages treated with 64 µg/mL of aqueous Quercus infectoria gall extract. The absorbances observed for TH17-related cytokines (IL-6, IL-13, IL-17A, IL-23, TNF-α, and TGF-β1) are displayed compared to those observed for untreated macrophages.
Nitric oxide (NO) production was determined using a Griess reaction. Macrophage cells were placed into wells of a 24-well plate (1 × 105 cells/ well), which then received the aqueous extract (64 µg/mL, 32 µg/mL, and 16 µg/mL); control wells received medium only. After 72 hours of incubation at 37 °C, the cell-free culture medium was removed from each well. This material (100 μL) was then combined with an equal volume of Griess solution (1% sulfanilamide, 0.1% naphthyl ethylenediamine in 5% phosphoric acid) and incubated at room temperature for 10 minutes. Thereafter, the absorbance was measured at 570 nm in the plate reader, and concentrations of NO were calculated by extrapolation from a standard curve prepared in parallel using sodium nitrite standards. These experiments were performed in triplicate.
A cytokine assay was performed according to the manufacturer’s instructions (multi-analyte ELISArray kit QIAGEN, USA) for the detection of murine macrophage TH1 (IL-2, IL-12, TNF-α, IFN-γ), TH2 (IL-4, IL-5, IL-6, IL-10, IL-13), and TH17 (IL-17A, IL-23, TGF-β1) cytokines. In this assay, 5 × 104 cells/mL cells were seeded in a 96-well plate and incubated overnight before treatment with 64 µg/mL concentration of extract. After 48 hours incubation, the culture supernatant was collected and assayed using a 96-well plate. After adding the DMSO, the color changed from blue to yellow, and the absorbance was measured at 450 nm within 30 minutes of stopping the reaction.
Statistical analysis was performed using the SPSS Statistics (IBM Corp. Released 2013. IBM SPSS Statistics for Windows, Version 22.0. Armonk, NY: IBM Corp.). Data obtained from at least three independent experiments are presented as the mean ± standard error (SE). Statistical evaluation of the results was performed by an independent t-test for proliferative and phagocytic assays and one-way ANOVA followed by a post-hoc Bonferroni test for the NO assay. Flow cytometry data were analyzed using FCS Express 6 Plus Research Edition (De Novo) software. A p-value < 0.050 was considered significant for all analyses.
Results
An increase in macrophage proliferation was observed in extract-treated cells compared to untreated macrophages for all tested extract concentrations [Figure 1]. The maximum percentage of proliferation was recorded at the concentration of 64 μg/mL at 72 hours with a percentage proliferation rate of 154.2±0.1%.
Flow cytometry analysis [Figure 2] visualized perturbations in phagocytosis of the cell population. The ability of the activated J774A.1 cells to phagocytose foreign particles was tested using fluorescent IgG-coated latex beads. Macrophages treated with Q. infectoria gall extract or PHA were expanded to the right lower quadrant. In contrast, the unstained macrophages were in the left lower quadrant of the dot plot, indicating that a macrophage increased its size during phagocytic activity. Flow cytometry analysis showed that without any treatment, only 55.1% of the cells phagocytosed the beads, while in macrophages treated with 16, 32, and 64 µg/mL of extract, the phagocytic activity increased to 60.8%, 60.9%, and 74.2%, respectively. However, the activity was not significantly different compared to the negative control. Phagocytosis was higher for macrophages treated with PHA (92.6%) compared to extract-treated macrophages [Figure 3].
The results of NO production by macrophages treated with three different extract concentrations are illustrated in Figure 4. A significant increase in NO production was observed for all treated groups in comparison to the untreated group. In contrast, cells treated with the highest concentration of extract (64 µg/mL) exhibited the lowest NO production [Figure 4].
The absorbance values of treated macrophages showed higher levels of IL-2, TNF-α, IL-5, IL-10, IL-13, IL-23, TGF-β1, and IL-17A, while lower values were observed for IL-12, IL-4, and IL-6. Overall, cytokine production by treated and untreated macrophages were statistically insignificant. The difference in the level of IL-13 produced by the treated and untreated macrophages was statistically significant [Figures 5, 6, and 7].
Discussion
The benefits of ethnomedicine cannot be ignored. For residents of developing countries, traditional medicine is preferred and can ultimately be more effective when providing aid. Recent studies show that traditional medicine is much more beneficial than once realized and may be an important aspect in the future of medicine.20 The present study examined the aqueous Q. infectoria gall extract for its effects on J774.1A macrophage cell viability to exclude any probable cytotoxic effects. The gall extract appears to be non-toxic to the cells and is associated with the proliferation of macrophages [Figure 1]. In a previous study, rectal administration of the aqueous extract showed low toxicity in rats.21
The role of macrophages in inflammation is indisputable. They are one of the key players in the initiation, development, and termination of inflammatory processes. The enhanced activity and proliferation of macrophages in the immune system depends not only on activation by a pathogen but can also be due to the modulation of exogenous active compounds in the gall extract, which possess anti-oxidant properties.22 Macrophages could undergo rapid in situ proliferation to increase population density in the absence of any intruders in the tissue.23 The proliferation of macrophages is controlled by cytokine IL-4, which is dominant in TH2 inflammation.
Phagocytosis is one of the essential mechanisms in the inflammatory process. Phagocytic cells of the innate immune system accumulate in large numbers within the affected tissue or site of injury and restore tissue homeostasis. Macrophages not only phagocytose and kill infectious or foreign agents but are also important in clearing dead cells and restoring or mediating tissue remodeling during the late stages of inflammation.24 In this study, the phagocytic activity of macrophages increased in a dose-dependent manner following the gall extract treatment. However, it was not as high as the activity produced by PHA-treated cells [Figure 3]. Furthermore, the extract-treated cell size was larger than the size of untreated macrophages, as shown in the flow cytometry analysis [Figure 2]. Another study also reported significant increased phagocytic activity and protective capacity of Q. infectoria gall extract against tert-butyl hydroperoxide stimulated rat peritoneal macrophages.5 The proliferation of macrophages also contributes to the enhancement of phagocytosis. Macrophages have the capacity to kill microbes and tumor cells after an activation process by increasing their size, producing more pseudopods, and disrupting the plasma membrane of
engulfed cells.25
NO is an important biological mediator and regulator of inflammation. In this study, extract-treated macrophages displayed a significant increase in NO production compared to untreated cells. Interestingly, the production of NO decreased in a dose-dependent manner, indicating that a higher concentration of gall extract would suppress NO generation [Figure 4]. This finding further supports the idea that Q. infectoria has anti-inflammatory properties. The extract inhibits various functions of macrophages and neutrophils related to the inflammatory response, including NO synthesis. Inflammatory macrophages constantly express inducible NO synthase (iNOS) to produce a large amount of NO. Q. infectoria gall extract is not only capable of inhibiting the generation of NO in macrophages, but also effectively scavenges free radicals induced by oxidative stress.4,26
TH1 cytokines (IFN-γ and IL-2) are usually predominant in innate immunity, typically against intracellular bacteria and protozoa, whereas TH2 cytokines play an important role in allergic disease and are typically against extracellular parasites, including helminths. The TH2 effector cytokines are IL-4, IL-5, IL-6, IL-10, IL-13, and IL-25. This study has demonstrated that the extract could reduce IL-4, IL-6, and IL-12 gene expression. These cytokines are important in the inflammatory process. IL-4 is a prototypic immunoregulatory cytokine. Like many cytokines, it can affect a variety of target cells in multiple ways. IL-4 has an important role in regulating antibody production, hematopoiesis and inflammation, and the development of effector T-cell responses. When acting on macrophages (a cell type critically involved in inflammation), IL-4 induces alternative macrophage activation, which acts as an anti-inflammatory and stimulates normal tissue repair.27
IL-6 is a proinflammatory cytokine involved in the generation and propagation of inflammation. It is also involved in acute phase reactions and is important in the development of specific cellular and humoral immune responses, including end-stage B cell differentiation, immunoglobulin secretion, and T-cell activation.28 In addition, IL-6 is the main switch in the transition between acute and chronic inflammation and a key factor in the recruitment of monocytes to the area of inflammation. IL-12 plays an important role in the activities of natural killer cells and T lymphocytes. As IL-12 is involved in TH1 development, it is believed to represent an important link between innate and adaptive immunity.29
This study indicated that Q. infectoria gall extract has anti-inflammatory potential. TNF-α cytokine levels increased due to treatment in contrast to results reported in another study in which TNF-α decreased significantly in extract-treated macrophages.5,30 Increases in cytokine levels (IL-2, IL-5, IL-10, IL-17A, IL-23, TGF-β1) were observed in this investigation but were not statistically significant. This study showed that IL-13 synthesis was significantly higher in extract-treated cells compared to a control. IL-13 has a role in inhibiting proinflammatory cytokines and chemokine production in vitro. It also acts as an anti-inflammatory cytokine, playing a unique role in the induction and maintenance of IgE production.29 TGF-β1 is a multifunctional cytokine involved in cell growth and differentiation.31 In the presence of IL-6, TGF-β1 drives the differentiation of TH17 cells. This action promotes further inflammation and increases autoimmune conditions.32 TH17 is a major helper cell involved in mucosal immunity and inflammation. It produces IL-17 and other cytokines that are good at fighting extracellular pathogens and fungi.
Conclusion
The extract of Q. infectoria gall is non-toxic and has a potential role in enhancing the proliferation and phagocytosis of macrophages, reducing NO production in a dose-dependent manner, and regulating cytokine levels in macrophages. This extract can modulate the inflammatory mode of macrophages via a reduction in iNOS and NO levels, as well as IL-4, IL-6, and IL-12 cytokines, while enhancing the production of IL-13 and other cytokines. Further detailed studies are required to identify the active constituents and their mechanisms for this effect both in vitro and in vivo.
Disclosure
The authors declared no conflicts of interest. The study was funded by the Universiti Sains Malaysia RU grant 1001/PPSK/812163.
Acknowledgements
We are grateful to the cell culture laboratory staff of the School of Health Sciences, Universiti Sains Malaysia, Malaysia for their support and technical assistance.
references
- 1. Male D, Brostoff J, Roth D, Roitt I. Immunology. 8th ed. Mosby: Elsevier Saunders; 2013.
- 2. Spiering MJ. Primer on the immune system. Alcohol Res 2015;37(2):171-175.
- 3. Kumar SV, Kumar SP, Rupesh D, Nitin K. Immunomodulatory effects of some traditional medicinal plants. J Chem Pharm Res 2011;3(1):675-684.
- 4. Kaur G, Hamid H, Ali A, Alam MS, Athar M. Antiinflammatory evaluation of alcoholic extract of galls of Quercus infectoria. J Ethnopharmacol 2004 Feb;90(2-3):285-292.
- 5. Kaur G, Athar M, Alam MS. Quercus infectoria galls possess antioxidant activity and abrogates oxidative stress-induced functional alterations in murine macrophages. Chem Biol Interact 2008 Feb;171(3):272-282.
- 6. Grieve M. A modern herbal: the medicinal, culinary, cosmetic and economic properties, cultivation and folk-lore of herbs, grasses, fungi, shrubs, & trees with all their modern scientific uses. Courier Corporation. 1971.
- 7. Basri DF, Fan SH. The potential of aqueous and acetone extracts of galls of Quercus infectoria as antibacterial agents. Indian J Pharmacol 2005;37(1):26-29 .
- 8. Yamunarani K, Jaganathan R, Bhaskaran R, Govindaraju P, Velazhahan R. In vitro antifungal activity of a 29-kDa glycoprotein purified from the galls of Quercus infectoria. Acta Phytopathol Entomol Hung 2005;40(1-2):43-54 .
- 9. Chusri S, Voravuthikunchai SP. Quercus infectoria: a candidate for the control of methicillin-resistant Staphylococcus aureus infections. Phytother Res 2008 Apr;22(4):560-562.
- 10. Vermani A; Navneet; Prabhat. Screening of Quercus infectoria gall extracts as anti-bacterial agents against dental pathogens. Indian J Dent Res 2009 Jul-Sep;20(3):337-339.
- 11. Haghi G, Safaei A. Identification and determination of polyphenols and tannin in the galls and in the extract of Quercus infectoria. Iran J Pharm Res 2010;(Suppl 2):85-86.
- 12. Basri DF, Tan LS, Shafiei Z, Zin NM. In vitro antibacterial activity of galls of Quercus infectoria olivier against oral pathogens. Evid Based Complement Alternat Med 2012;2012:632796.
- 13. Hassan HF. Study the effect of Quercus infectoria galls extracts on growth of Candida albicans and Candida glabrata in vitro which isolated from vaginal swabs. Iraqi J Vet Sci 2011;35(2):85-94 .
- 14. Hassan HF. Isolation and identification of oral Candida sp. from leukemic children under chemotherapy and treatment with extraction of different plants in vitro. Dentistry 2012;24(4):152-156.
- 15. Hashim ST. Bacteriological and biochemical study for effect of phenolic extract of Quercus infectoria against some food-born pathogenic bacteria. Indian J Appl Res 2013;3(7):52-55 .
- 16. Rao N, Mittal S, Sudhan S, Menghani E. In vitro phytochemical screening, antioxidant and antimicrobial activity of the methanolic extract of Quercus Infectoria L. Int J Pharm Pharm Sci 2013;5(2):273-277.
- 17. Baharuddin NS, Abdullah H, Abdul Wahab WN. Anti-Candida activity of Quercus infectoria gall extracts against Candida species. J Pharm Bioallied Sci 2015 Jan-Mar;7(1):15-20.
- 18. Asif M, Ansari SH, Haque MR, Kalam N. Estimation of total phenolic, flavonoid contents and antioxidant activity in the nut galls of Quercus infectoria Olivier. J Pharm Res 2012;5(7):3855-3857.
- 19. Ansari AM, Haque MR, Kalam N. Standardization and contamination studies on nutgalls of Quercus infectoria olivier. International Research Journal of Pharmacy 2012;3:149-152.
- 20. Divakar MC, Al-Siyabi A, Varghese SS, Rubaie MA. The practice of ethnomedicine in the northern and southern provinces of Oman. Oman Med J 2016 Jul;31(4):245-252.
- 21. Iminjan M, Amat N, Li XH, Upur H, Ahmat D, He B. Investigation into the toxicity of traditional Uyghur medicine Quercus infectoria galls water extract. PLoS One 2014 Mar;9(3):e90756.
- 22. Chokpaisarn J, Chusri S, Amnuaikit T, Udomuksorn W, Voravuthikunchai SP. Potential wound healing activity of Quercus infectoria formulation in diabetic rats. PeerJ 2017 Jul;5:e3608.
- 23. Jenkins SJ, Ruckerl D, Cook PC, Jones LH, Finkelman FD, van Rooijen N, et al. Local macrophage proliferation, rather than recruitment from the blood, is a signature of TH2 inflammation. Science 2011 Jun;332(6035):1284-1288.
- 24. Helming L. Inflammation: cell recruitment versus local proliferation. Curr Biol 2011 Jul;21(14):R548-R550.
- 25. Fujiwara N, Kobayashi K. Macrophages in inflammation. Current drug target-inflammation and allergy 2005;4(3):281-286.
- 26. Hamid H, Kaur G, Abdullah ST, Ali M, Athar M, Alam MS. Two new compounds from the galls of Quercus infectoria with nitric oxide and superoxide inhibiting ability. Pharm Biol 2005;43(4):317-323.
- 27. Luzina IG, Keegan AD, Heller NM, Rook GA, Shea-Donohue T, Atamas SP. Regulation of inflammation by interleukin-4: a review of “alternatives”. J Leukoc Biol 2012 Oct;92(4):753-764.
- 28. Gabay C. Interleukin-6 and chronic inflammation. Arthritis Res Ther 2006;8(Suppl 2):S3.
- 29. Foster JR. The functions of cytokines and their uses in toxicology. Int J Exp Pathol 2001 Jun;82(3):171-192.
- 30. Lodhi G, Singh H, Pant K, Rao CV, Hussain Z. Hepatoprotective effects of Quercus infectoria gall extract against carbon tetrachloride treated liver injury in rats. Int J Appl Res Nat Prod 2012;5(3):17-22.
- 31. Jin Y, Wi HJ, Choi MH, Hong ST, Bae YM. Regulation of anti-inflammatory cytokines IL-10 and TGF-β in mouse dendritic cells through treatment with Clonorchis sinensis crude antigen. Exp Mol Med 2014 Jan;46(1):e74.
- 32. Korn T, Bettelli E, Oukka M, Kuchroo VK. IL-17 and Th17 cells. Annu Rev Immunol 2009;27:485-517.